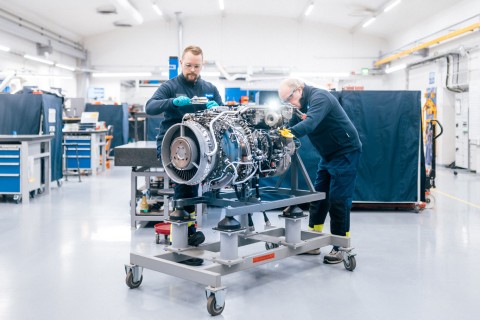
Patria (formerly Valmet) launched its pioneering work in digital avionics back in the early 80s. By 1983, the company was modernising eastern aircraft with western avionics and had already begun to produce its own microprocessor-based devices. This created a foundation for projects to build a data link system for the Draken fleet. When Finland was taking its first steps to renew its fighter fleet, we were more prepared to take responsibility for developing aircraft equipment for the F/A-18 Hornet’s national data link system, and later for building the Hornet’s system testing environment (the Software Test and Integration Center – STIC).
Text and photos: Eero Kovamäki
Thanks to our advanced level of expertise, we were able to implement a major cockpit update to the Hawk fleet, starting in 2007 with the Hawk Mk.51 fleet and continuing with modifications to Mk.66 aircraft acquired from Switzerland. We then updated another seven Mk.51 aircraft. All in all, a total of 31 Hawk training fighters were given a complete overhaul. Finland acquired the competence to maintain the Hawk’s mission computer software during the first stage of the project.
Patria engineers were trained in the USA, and the source code acquired during the first-phase contract negotiations ensured that we could make continual developments to the mission computer. The capability to engage in local further development close to our end-users in Finland guaranteed high performance for the Hawk fleet far into the future.
The integration experience gained from the Hawk project has given Patria the ability to provide similar services for other fleets, two prime examples being the Finnish Border Guard’s Bell 412 project and GROB modifications for the Finnish Air Force.
The almost completely upgraded Hawk cockpit was given some familiar elements from the Hornet:
- MDF – Multi Function Display
- HUD – Head-Up Display
- UFCP – Up-Front Control Panel
- MDR – Mission Data Recorder (for importing and exporting data)
- NAV/COM/DME/VOR/ILS (redesigned radio and navigation equipment with a digital bus interface)
- INS/GPS locationing system
- ADC – air data computer with digital bus interface (speed and altitude data).
What does avionics mean?
The word avionics is a composite of two words: aviation and electronics.
Generally speaking, you could say that all the electronic systems used in aircraft are avionics systems. However, some military aircraft manufacturers use the term avionics to refer to traditional flight systems, such as communication radio and navigation systems. Military electronics, such as radar, are referred to as weapon systems.
Useful payloads, such as searchlights and cameras, are sometimes called avionics and sometimes called mission systems.
Avionics can be further divided in several ways, such as analogue and digital. Nowadays, with the digitalisation of technology in general, computer-based systems have gained ground from analogue systems in aviation electronics as well.
The avionics required for basic flight can be extremely simple when you think of, for example, a glider. But small planes are typically equipped with a communication system, navigation system and flight status system (altitude, speed, roll, pitch and yaw). The aircraft’s power plant – the engine – also needs its own sensors and meters to monitor functionality.
What are avionics in practice, and what do they consist of in a typical military aircraft?
In a typical military aircraft, avionics are widely understood to refer to all of the electronics that are involved in flying the plane. These systems are usually grouped as follows:
- Communication, Radio Navigation, Identification (CNI)
- Navigation and Flight Aids (such as GPS/INS, Air Data Computer)
- Status Monitoring Equipment
- Flight and Mission Recorders
- Mission Computers
- Tactical Sensors (such as Radar, FLIR)
- Stores Management Systems (Armament Computer, Decoders, Pylons)
- …
All of the aforementioned groups consist of several devices that are connected in various different ways; these days most typically with digital data buses.
Mission Computers are an aircraft’s most important seat of intelligence, and are usually duplicated for safety. If one device is damaged, the other will be able to handle critical functions.
These days, the displays in military aircraft are typically digital LCD multifunction displays. Although touch screens are used, it is more important for pilots to keep their hands on the power lever and joystick, which are full of switches for various functions. (HOTAS – Hands On Throttle And Stick).
Because there are so many sensors and systems, software is used to combine data from all the various sensors into a clear picture of the situation, in order to reduce the pilot’s workload and facilitate situational awareness (SA).
Radar has traditionally been the most important of an aircraft’s tactical sensors, but it now works alongside optical devices – systems that utilise infrared and lasers.
Navigation has become easier with the advent of map display systems and GPS. Inertial Navigation Systems (INS) have also become more accurate and advanced, so that the pilot is always aware of the aircraft’s flight status.
Digitalisation
Since digitalisation, aircraft systems have been able to precisely monitor their own condition and relay their status to both the pilot’s display and the recorder.
The recorder plays many roles. Missions are typically prepared on ground systems (Mission Planning Systems) before take-off, and the plan is then imported into the aircraft using a memory device, for example, onto a map template on the pilot’s display. During the flight, information is collected about the route, the various stages of the tactical mission, the condition of the equipment, the pilot’s flight time, and so on. After the aircraft has landed, this information is stored in the ground systems so that flights made by several aircraft during joint exercises can be examined in the Mission Debriefing System.
The communication systems in military aircraft typically include not only a voice connection but also digital data transfer with other aircraft and between the aircraft and the combat leader on the ground (a data link). This data connection is usually encrypted and protected, so that messages will get through even during intentional interference. The voice connection is often digital and likewise protected from intentional interference.
A military aircraft employs a variety of sensors to detect the opponent and expose it to the aircraft’s weaponry. The aircraft tries to avoid the opponent using its Electronic Warfare (EW) systems. Typical devices include systems that release metal chaff to protect the aircraft from radar-guided missiles, or systems that throw flares to protect the aircraft from heat-seeking missiles. Radio frequency jammers will also be used to interfere with the opponent’s radar. These jammers usually operate automatically in a well-researched and accurately defined manner when the aircraft’s Radar Warning Receiver (RWR) alarms.
What are the most important components/systems?
The aforementioned computers and their software have assumed a central role in military aircraft systems. Almost every device contains at least a microprocessor that controls its own functionality, continually monitors its own condition, and communicates directly with the mission computer or device cluster’s central processor via digital data buses. The radar system, for example, will consist of numerous devices that are located in various cabinets all over the plane. Likewise, the Stores Management System (SMS) is a weapon system computer that communicates with both the mission computer and its own subunits. The same goes for the Electronic Warfare system’s devices. Along with their own digital data connections, they each form their own local network.
As sensor systems have become more complex and diverse, the pilot would be subjected to an unbearable flood of information without software to combine the sensor data. With the aid of a Multi Sensor Integration (MSI) algorithm, sensor and data fusion is employed to determine the best value from all the data available about an object from different sources. This enables the clearest and most accurate detection of a particular object.
A dual-redundant MIL-STD-1553B data bus has long been the standard for data transfer in military aircraft, as it has been designed to work in spite of interference. The aim has been to achieve reliable data transfer between devices, even when some of the device connections have been damaged. In the latest aircraft models, this data bus is starting to slow down due to more advanced sensors that must transfer a lot of video footage to the recorder or the pilot’s screen. However, flight-critical data transfer using the 1553B data bus continues to hold its ground.
When building new avionics, it is important to bear in mind the demanding environmental requirements – not only during performance, but also in terms of the aircraft’s long service life and the maintenance of a spare parts inventory.
Military aircraft usually wrestle with the following difficult requirements:
- ambient temperature (can range between –55 and +125°C)
- impacts (such as landing on an aircraft carrier or at a road base)
- acceleration (including collision safety)
- pressure (items such as capacitors and display components must be chosen carefully)
- rapid pressure variations (if, for example, the canopy breaks)
- electromagnetic compatibility (EMC, interference tolerance, cannot itself cause interference)
- the power supply’s compatibility with variable (‘raw’) electricity generated by the aircraft
- explosive atmospheric conditions (due to fuel vapours)
- …
These environmental factors pose challenges for the design and manufacture of new devices and adapters, and also for verifying compliance with requirements.
In addition to the environmental requirements for hardware, there are certain distinctive features associated with producing aviation software. Software quality must be achieved using a process-based approach and not through “field testing”. For example, the DO-178 standard development model specifies different levels of software criticality. Each criticality level defines the test cases and reviews that must be performed on the software.